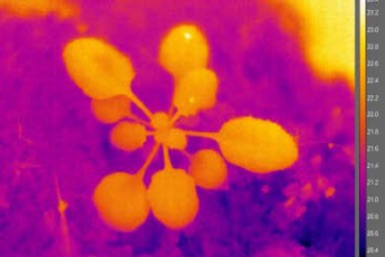
Biologists have described a new molecular mechanism that allows plants to optimize their growth under suboptimal high-temperature conditions.
Biologists have described a new molecular mechanism that allows plants to optimize their growth under suboptimal high-temperature conditions.
Researchers have revealed the role of genes in controlling flowering time in the Brassica rapa family. They demonstrated that a higher level of FLC gene expression is essential for inhibiting flowering in the absence of a cold period and also discovered that the rate of repression of FLC expression during a cold exposure affects the time of flowering. It is hoped that this understanding can contribute to the efficiency of B. rapavegetable cultivation in the face of climate change.
Quinoa is a healthy food many know and love. As its popularity grows, more farmers are interested in planting it. However, the plant doesn’t do well in high temperatures, so plant breeders are trying to help.
Research combining future climate conditions and arsenic-induced soil stresses predicts rice yields could decline about 40 percent by 2100, a loss that would impact about 2 billion people dependent on the global crop.
The intensity of summer algal blooms has increased over the past three decades, according to a first-ever global survey of dozens of large, freshwater lakes.
Scientists identified significant new chromosomal regions for wheat yield and disease resistance, which will speed up global breeding efforts.
Using the full wheat genome map published in 2018, combined with data from field testing of wheat breeding lines in multiple countries, an international team of scientists has identified significant new chromosomal regions for wheat yield and disease resistance and created a freely-available collection of genetic information and markers for more than 40,000 wheat lines.
Reported in Nature Genetics, the results will speed up global efforts to breed more productive and climate-resilient varieties of bread wheat, a critical crop for world food security that is under threat from rising temperatures, rapidly-evolving fungal pathogens, and more frequent droughts, according to Philomin Juliana, wheat scientist at the International Maize and Wheat Improvement Center (CIMMYT) and first author of the new study.
“This work directly connects the wheat genome reference map with wheat lines and extensive field data from CIMMYT’s global wheat breeding network,” said Juliana. “That network in turn links to over 200 breeding programs and research centers worldwide and contributes to yield and other key traits in varieties sown on nearly half the world’s wheat lands.”
The staple food for more than 2.5 billion people, wheat provides 20% of human dietary calories and protein worldwide and is critical for the nutrition and food security of hundreds of millions of poor persons in regions such as North Africa and South Asia.
“Farmers and societies today face new challenges to feed rising and rapidly-urbanizing populations, and wheat epitomizes the issues,” said Ravi Singh, CIMMYT wheat breeder and corresponding author of the study. “Higher temperatures are holding back yields in major wheat-growing areas, extreme weather events are common, crop diseases are spreading and becoming more virulent, and soil and water are being depleted.”
Juliana said the study results help pave the way to apply genomic selection, an approach that has transformed dairy cow husbandry, for more efficient wheat breeding.
“Molecular markers are getting cheaper to use; meanwhile, it’s very costly to do field testing and selection involving many thousands of wheat plants over successive generations,” Juliana said. “Genome-wide marker-based selection can help breeders to precisely identify good lines in early breeding generations and to test plantlets in greenhouses, thereby complementing and streamlining field testing.”
The new study found that genomic selection could be particularly effective in breeding for wheat end-use quality and for resistance to stem rust disease, whose causal pathogen has been evolving and spreading in the form of highly-virulent new races.
The new study also documents the effectiveness of the global public breeding efforts by CIMMYT and partners, showing that improved wheat varieties from this work have accumulated multiple gene variants that favor higher yields, according to Hans-Joachim Braun, director of CIMMYT’s global wheat program.
“This international collaboration, which is the world’s largest publicly-funded wheat breeding program, benefits farmers worldwide and offers high-quality wheat lines that are released directly to farmers in countries, such as Afghanistan, that are unable to run a full-fledged wheat breeding program,”
Braun explained.
The study results are expected to support future gene discovery, molecular breeding, and gene editing in wheat, Braun said.
Together with more resource-efficient cropping systems, high-yielding and climate-resilient wheat varieties will constitute a key component of the sustainable intensification of food production described in Strategy 3 of the recent EAT-Lancet Commission recommendations to transform the global food system. Large-scale genomics will play a key role in developing these varieties and staying ahead of climate- and disease-related threats to food security.
Read the paper: Nature Genetics
Article source: CIMMYT
Image: Apollo Habtamu/CIMMYT
Back in the 1960s, when the Green Revolution started, the need was to provide calories for a starving world. Today, food is cheap. But it comes at great environmental cost. Climate change and the need to feed an ever-growing world population in a healthy way without ruining the natural environment are inextricably linked. It’s hard to see how to solve such “wicked problems”. The authors show that a dramatic change in the human diet could make a huge difference.
This week we spoke to Dr. Joe Cornelius, the Program Director at the Advanced Research Projects Agency – Energy (ARPA-E). His work focusses on bioenergy production and conversion as a renewable and sustainable energy source, transportation fuel, and chemical feedstock, applying innovations in biotechnology, genomics, metabolic engineering, molecular breeding, computational analytics, remote sensing, and precision robotics to improve biomass energy density, production intensity, and environmental impacts.
What is ARPA-E? How are programs created?
The Advanced Research Projects Agency-Energy (ARPA-E) is a young government agency in the U.S. Department of Energy. The agency is modeled on a successful Defense Department program, the Defense Advanced Research Projects Agency (DARPA). Both agencies target high-risk, high-reward research in early-stage technologies that are not yet ready for private-sector investment.
Program development is one of the unique characteristics of the agency. ARPA-E projects are in the hands of term-limited program directors, who develop a broad portfolio of concepts that could make a large impact in the agency’s three primary mission areas: energy security, energy efficiency, and emissions reductions. The agency motto is “Changing what’s possible”, and we are always asking ourselves, “if it works, will it matter?”. Getting a program approved is a lot like a doing a PhD; you survey the field, host a workshop, determine key points to research, define aggressive performance metrics, and finally defend the idea to the faculty. If the idea passes muster, the agency makes a targeted investment. This flexibility was recently noticed as one of the great aspects of ARPA-E culture and is an exciting part of the job.
What is TERRA and how is it new for agriculture?
TERRA stands for Transportation Energy Resources from Renewable Agriculture, and its impact mission is to accelerate genetic gains in plant breeding. This is an advanced analytics platform for plant breeding. Today, significant scientific progress is possible through the convergence of diverse technologies, and TERRA’s innovation for breeders comes through the integration of remote sensing, computer vision, analytics, and genetics. The teams are using robots to carry cameras to the field and then extracting phenotypes and performing gene linkages. It’s really awesome to see.
This is run by the U.S. Department of Energy. How does TERRA tie into energy?
The United States has a great potential to generate biomass for conversion to cellulosic ethanol, but the crops useful for producing this biomass have not seen the improvement that others, such as soybeans or maize, have had. TERRA is focused on sorghum, which is a productive and resilient crop with existing commercial infrastructure that can yield advanced biomass on marginal lands. In addition, sorghum is a key food and feed crop, and the rest of the world will benefit from these advancements.
How does TERRA address the challenge of phenotyping in the field?
The real challenges that remain are in calibrating the sensor output and generating biological insight. A colleague from the United Kingdom, Tony Pridmore, captured the thought well, saying “Photography is not phenotying.” It’s generally easy to take the pictures — unless it’s very windy, the aerial platforms can pass over any crop, and the ground platforms are based on proven agricultural equipment. To get biological insights however, each team requires an analytics component, and a team from IBM is contributing their analytics expertise in collaboration with Purdue University.
What is most exciting about the TERRA program?
We commissioned the world’s biggest agricultural field robot, which phenotypes year-round. The six teams have successfully built other lightweight platforms involving tractors, rovers, mini-bots, and fixed and rotary wing unmanned aerial vehicles. It’s exciting to see some of the most advanced technologies move so quickly into the hands of great geneticists. The amazing thing is how quickly the teams have started generating phenotyping data. I expected it to take years before we got to this point, but the teams are knocking it out of the park, and we are entering into full-blown breeding systems deployment.
Who’s on the TERRA teams? How did you build the program?
ARPA-E system teams include large businesses, startups, and university groups. The program was built to have a full portfolio of diverse sensor suites, robotic platform types (ground and aerial), analytics approaches, and geographic breadth. Because breeders are working for a particular target population of environments, different phenotypes are valued differently across the various geographies. For that reason, each group is collecting its own set of phenotypes. Beyond that, we’ve worked very hard to encourage collaboration across the teams and have an exciting GxE (genotype x environment) experiment running, where several teams plant the same germplasm across multiple geographies. By combining this with high-throughput phenotyping, the teams are in a good position to determine key environmental inputs to various traits.
Once we achieve rapid-fire field phenotyping, what’s next?
We’re going underground! ARPA-E has made another targeted investment, this time in root phenotyping. We’re really excited about this one. It’s a very similar concept, but the sensing is so much harder. The teams have collaborated with medical, mining, aerospace, and defense communities for technologies that can allow us to observe root and soil systems in the field to allow breeders to improve crops. Ask us again next year—we will have some cool updates to both programs!
This article was republished from SciDev.Net.
Relocating coffee areas, along with forestation and forest conservation, to higher altitudes to cope with climate change could increase Ethiopia‘s coffee farming area fourfold, a study predicts.
The study, published in Nature last month (19 June), suggests that moving Ethiopian coffee fields to higher ground because of climate change could increase resilience by substantially increasing the country’s suitable production area.
Justin Moat, spatial analyst at the UK’s Royal Botanic Gardens Kew, and lead author of the study, says that currently coffee farming is mainly confined to altitudes between 1200 and 2200 metres.
“A critical factor in the suitability of coffee farming is the interaction between rainfall and temperature.”
Justin Moat
“In general, coffee’s niche will move uphill to keep to optimal temperature,“ he tells SciDev.Net. “Much work would be needed to achieve this if planning starts now.”
According to Moat, up to 60 per cent of the country‘s current production area could become unsuitable before the end of the century.
Ethiopia, he says, is the world’s 5th largest coffee producer. The crop provides a quarter of export earnings, and approximately 15 million Ethiopians engage in coffee farming and production.
The study‘s results were based on computer modelling and simulations. “We determined coffee-preferred climate (niche) using a huge amount of data collected on the ground, including historic observations, overlaid on climate maps,” explains Moat.
They projected this niche into the future using climate models and scenarios, which revealed that all the models were in general agreement. They then combined this with satellite imagery to come up with the present-day forest coffee area, and the area projected in the future.
Higher altitudes are forecast to become more suitable for coffee while lower altitudes are projected to become less suitable, according to the study.
“A critical factor in the suitability of coffee farming is the interaction between rainfall and temperature; higher temperatures could be tolerated if there was an increase in rainfall,” Moat notes.
He adds that regardless of interventions, one of the country‘s best known coffee-growing regions — Harar, in eastern Ethiopia — is likely to disappear before the end of the century.
Shem Wandiga, a professor of chemistry at the University of Nairobi’s Institute for Climate Change Adaptation, Kenya, says that although the study cannot predict with full certainty, it holds important messages for policymakers.
“Start planning to expand coffee growing areas to higher elevation, he suggests. “The expansion should be coupled with forestation of the areas.“
Researchers and policymakers should also map out the human, social and ecological conditions that may allow such expansion, according to Wandiga. Also, farmers should slowly substitute coffee with other plants that may bring income.
William Ndegwa, Kitui County director at the Kenya Meteorological Department, says the model used in the research is a powerful tool for linking climate variables with biological parameters.
“This is a very interesting [study] with deep insights into the characteristics of the impacts of climate change on crop production,” he notes.
This piece was produced by SciDev.Net’s Sub-Saharan Africa-English desk.
This article was originally published on SciDev.Net. Read the original article.
Professor Henk Hilhorst (Wageningen University and Research)
This week we spoke to Professor Henk Hilhorst (Wageningen University and Research) about his research on desiccation tolerance in seeds and plants.
Could you begin by telling us a little about your research?
I am a plant physiologist specializing in seed biology. I have a long research record on various aspects of seeds, including the mechanisms and regulation of germination and dormancy, desiccation tolerance, as well as issues in seed technology. Being six years from retirement now, I decided to extend my desiccation tolerance studies from seeds to resurrection plants, which display vegetative desiccation tolerance. I strongly believe that unveiling of the mechanism of vegetative desiccation tolerance may help us create crops that are truly tolerant to severe drought, rather than (temporarily) resistant.
How did you become interested in this field of study, and how has your career progressed?
As with many things in life, it was coincidence. I majored in plant biochemistry and applied for a PhD position in seed biology. After obtaining the degree I was offered a tenure track position in seed physiology by the Laboratory of Plant Physiology at Wageningen University, where I still work as a faculty member. My career has progressed nicely and I am an authority in the field of seed science, editor-in-chief of the journal Seed Science Research, and will become the President of the International Society for Seed Science in September of this year.
I see my current work on vegetative desiccation tolerance as a highlight in my professional life. I have always been more interested in the desiccation tolerance of seeds until about five years ago, when my current collaborator Prof Jill Farrant of the University of Cape Town, South-Africa, made me enthusiastic about these wonderful resurrection plants. We started to work together and published our first study recently in Nature Plants.
Read the paper here ($): A footprint of desiccation tolerance in the genome of Xerophyta viscosa.
In your recent paper, you sequenced the genome of the resurrection plant, Xerophyta viscosa, which can survive with less than a 5% relative water content. How is it possible for a plant to lose so much of its water and still survive?
These plants have a lot of characteristics that we’ve seen in seeds. They display protective desiccation tolerance mechanisms in their leaves, including anti-oxidants, protective proteins, and even dismantle their photosynthetic machinery during periods of drought. Even the cell wall structure and composition of resurrection plants resemble those of seeds. We are currently working on a paper describing the striking similarities between seeds and resurrection plants.
What was the most interesting discovery you made upon sequencing the genome of the resurrection plant?
First, the similarities between resurrection plants and seeds listed above were also apparent at the molecular level. For example, previous work suggested that the “ABI3 regulon”, consisting of about 100 genes regulated by the transcription factor ABI3, is specific to seeds, but we found that it is almost completely present (and active) in the leaves of Xerophyta viscosa too!
Secondly, we found “islands” or clusters of genes specific for desiccation tolerance that aren’t found in other species. Many of these regulate secondary metabolite pathways.
How challenging was it to sequence the genome of this plant? How did you overcome any difficulties?
It was very challenging. First, the species is an octoploid, meaning it has eight copies of each chromosome. This meant that we had to sequence its genome at very high coverage and employing the most advanced sequencing facilities, e.g. PacBio. Getting funding for this complex analysis was another challenge. We then took almost a year to assemble the genome and annotate it at the desired quality.
You identified some of the most important genes involved in desiccation tolerance. Is it possible to translate this work into other species, such as crops that may be threatened by drought as the climate changes?
That will be our ultimate goal. It’s important to remember that desiccation-sensitive plants, including all our major crops, produce seeds that are desiccation tolerant. This implies that the information for desiccation tolerance is present in the genomes of these crops but that it is only turned on in the seeds. We are trying to determine how this is localized, in order to find a method to turn on the desiccation tolerance mechanism in vegetative parts of the (crop) plant too. In parallel we are expressing some of the key transcription factors from Xerophyta viscosa in some important crops to see how this affects them.
Are there any other interesting aspects of Xerophyta viscosa biology?
Contrary to plants that wilt and ultimately die because of (severe) drought, leaves of resurrection species do not show such stress-related senescence. This is related to the engagement of active anti-senescence genes during the drying of the leaves of resurrection species. We are currently investigating these senescence-related mechanisms too.
The rose of Jericho (Anastatica hierochuntica) is another resurrection plant. Image credit: FloraTrek. Used under license: CC BY-SA 3.0.
Do you expect to find that different types of desiccation-tolerant plants use the same subset of genes to survive drought, or could they have developed other pathways to resilience?
We expect that the core mechanism is very similar among the resurrection species but that each species may have adapted to its specific environment.
Funding permitting, we will sequence the genomes of at least another ten resurrection species to further clarify the various evolutionary pathways to desiccation tolerance and, importantly, to discriminate between species-specific and desiccation tolerance-specific genes.
What advice do you have for early career researchers?
Stick to what you believe in, even if you have to (temporarily) be involved in research that you appreciate less, e.g., because of better funding opportunities.
Read Henk’s recent paper in Nature Plants here ($): A footprint of desiccation tolerance in the genome of Xerophyta viscosa.