This week we speak to Dr Nils Stein, Group Leader of the Genomics of Genetic Resources group at the Leibniz Institute of Plant Genetics and Crop Plant Research (IPK). We discuss his recent work on the genomes of 6000-year-old cultivated barley grains, published in Nature Genetics, which made the headlines around the world.
Could you describe your work with the Leibniz Institute of Plant Genetics and Crop Plant Research (IPK)?
The major research focuses of my group, the Genomics of Genetic Resources, are to continue sequencing the genomes of barley and wheat, perform comparative genomics on the Triticeae tribe, isolate genes of agronomic interest, and investigate the genomics of wild barley relatives.
We are currently leading the work to generate the barley reference genome, and we are also partners in several wheat genome sequencing projects. We are genotyping-by-sequencing (GBS) all 20 000 barley accessions in the IPK Genebank, as well as 10 000 pepper accessions as part of a Horizon 2020 project (G2P-SOL) investigating the Solanaceae crop species.
Your recent collaborative paper on the genomic analysis of 6,000-year-old barley grains made headlines around the world. What did this study involve?
This was an interdisciplinary study to sequence the DNA of 6000-year-old barley grains. The grains were excavated by a team of Israeli archaeologists and archaeobotanists led by Prof. Ehud Weiss, Bar-Ilan University, the DNA was extracted and sequenced by ancient DNA specialists Prof. Johannes Krause and Dr. Verena Schünemann in Germany, and the data were analyzed by Dr. Martin Mascher in the context of our comprehensive barley genome diversity information. This allowed the resulting sequence information to be put into a population genetic and ecogeographic context.

Preserved remains of rope, seeds, reeds and pellets (left), and a desiccated barley grain (right) found at Yoram Cave in the Judean Desert. Credit: Uri Davidovich and Ehud Weiss.
What led you to the realization that barley domestication occurred very early in our agricultural history?
The genome of the analyzed ancient samples was highly conserved with extant barley landraces of the Levant region, which look very similar to today’s high-yielding barley varieties. Although suggestive and tendentious, this told us that the barley crop 6000 years ago looked very similar to extant material. The physical appearance and the archaeobotanical characters of the analyzed seeds also very much resembled modern barley.
These barley grains contain the oldest plant genomes reconstructed to date. Did you find any differences between the samples that might give us an insight into the traits that were first selected in the early domestication of the crop?
We have only scratched the surface so far. The major domestication genes controlling dehiscence, brittleness or row-type of the main inflorescence had the same alleles in the ancient samples that are found in extant barley, confirming that these traits were selected for early in domestication. Additional analyses on other genes controlling different traits in barley are still ongoing – bear in mind that many of the genes controlling major traits in barley are still unknown, which complicates the selection of targets for analysis.
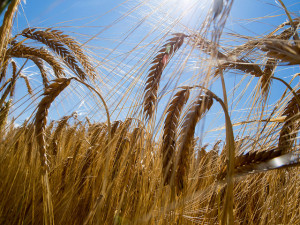
Modern barley cultivar. Credit: Christian Scheja. Used under license: CC BY 2.0.
Do these grains have any genetic variation that we lack at key loci in modern barley lines, for example in stress or disease resistance?
This is matter of ongoing analysis. So far it is obvious that the most genetically similar extant landraces from the Levant region have accumulated natural mutations over the last 6000 years, resulting in additional variation that we don’t find in the ancient sample.
What can we expect from the barley genome projects in the future?
The International Barley Genome Sequencing Consortium is preparing a manuscript on the reference sequence of barley. This will allow further analysis of the ancient DNA data with a more complete, genome-wide view, including the consideration of a more complete gene set than has been available so far. Our Israeli collaborators (Professor Ehud Weiss and Professor Tzion Fahima) have more ancient samples of similar quality. We hope we will be able to generate a more comprehensive view of the ancient population genomics of barley in the future, to better address the question of novel ancient alleles and lost genetic diversity.
The Barley Pan-Genome analysis will soon give us a better understanding of the structural variation in the barley genome. Putting the ancient DNA information into this more comprehensive genomic context will be very exciting. We also hope to be able to compare a variety of ancient samples of different ages to more precisely date the event of barley domestication.
You can read the paper here: Genomic analysis of 6000-year-old cultivated grain illuminates the domestication history of barley ($).