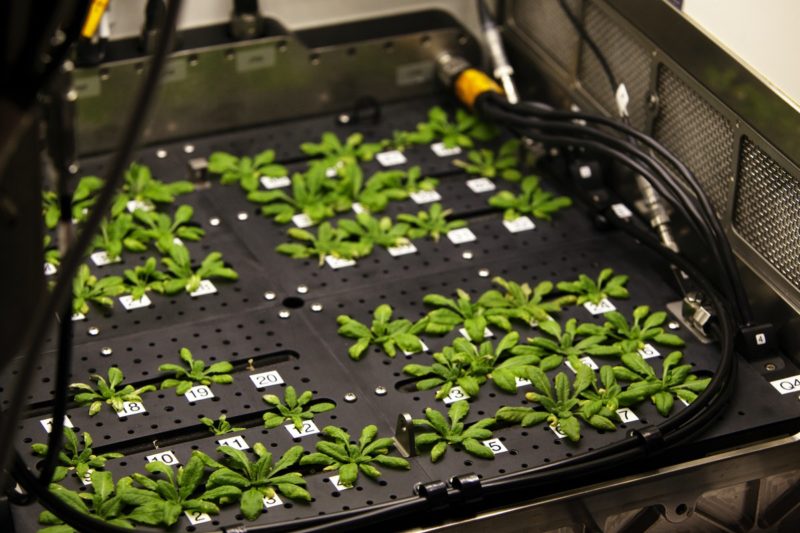
Scientists have helped find a way to control different plant processes – such as when they grow – using nothing but coloured light.
Scientists have helped find a way to control different plant processes – such as when they grow – using nothing but coloured light.
A research team’s model to explain photosynthesis lays out the next challenging phase of research on how green plants transform light energy into chemical energy
In the course of the COVID-19 pandemic, scientists are facing great challenges because they have to reorient, interrupt or even cancel research and teaching. International researchers demand the active protection and support of diversity, equity and inclusion in science.
Plants produce the hormone jasmonic acid as a defence response when challenged. This is how they ensure that their predators no longer like the taste of their leaves. Biologists want to find out whether biological precursors and other variants of jasmonic acid lead to similar or different effects. But such derivatives of the hormone have so far been too expensive for experiments and difficult to come by. Researchers have now found a method that might make the production of a biologically significant precursor of jasmonic acid more efficient and cheaper.
A group of scientists from Sechenov University, Russia, and La Trobe University, Australia, have developed a fast and cost-effective method of detecting and identifying bioactive compounds in complex samples such as plant extracts. They successfully applied the method to examine Mediterranean and Australian native culinary herbs. Three articles on this work were published in Applied Sciences, Journal of Pharmaceutical and Biomedical Analysis and Journal of Chromatography A.
Since ancient times, people have been using herbs as food additives and medicines, though a search for useful compounds and a study of their properties remain a difficult task. It is possible to examine a compound if it is stable enough and can be separated from other substances in a sample. However, plant extracts contain hundreds of compounds. In the past, only known compounds were investigated by target analysis and most bioactive compounds were left undiscovered. Thus, the number of compounds that are yet to be explored is so huge that methods that can both screen mixtures and identify the compounds responsible for bioactivity are of greater value.
The authors of the papers used an Effect Directed Analysis (EDA) approach, which is a combination of chromatographic separation with in situ (bio)assays and physico-chemical characterisation to discover and identify bioactive compounds in complex plant samples. Thin-layer chromatography (TLC) and high performance thin-layer chromatography (HPTLC) are well established, chromatographic separation techniques ideally suited for high-throughput screening of bioactive compounds in crude samples.
To separate substances, TLC uses the fact that various compounds are transported by a solvent and absorbed by a sorbent at different speeds. A sorbent-coated plate with a studied mixture is immersed with one end in the solvent, and under the action of capillary forces, it begins to rise along the plate, taking the substances of the mixture with it. As they move upward, the compounds are absorbed by the sorbent and remain as horizontal bands that can be distinguished in visible, infrared or ultraviolet light. Using this method, crude extracts can be analysed directly with no preparation and possible loss of sample components.
Bioassays allow to determine the properties of compounds, such as toxicity, observing how model organisms (bacteria, plants or small animals) react to them. In this way, one can select extracts able to inhibit the action of individual enzymes or reactive oxygen species.
Combination of TLC chromatography with microbial (bacteria and yeast) tests and biochemical (enzyme) bioassays enables rapid and reliable characterization of bioactive compounds directly on the chromatographic plates, without isolation/extraction. The advantage of HPTLC is that plates/chromatograms can be directly immersed into enzyme solution (bioassays), incubated for up to several hours, followed by visualization of the (bio)activity profile via an enzyme substrate reaction as bioactivity zones. This approach is more cost effective, enabling a more streamlined method to detect and characterise natural products that are suitable candidates for further investigation as potential new drug molecules.
Using this method, scientists examined the properties of bioactive compounds from culinary herbs commonly used in the Mediterranean diet: basil, lavender, rosemary, oregano, sage and thyme. Australia’s native plants were added to the list: lemon myrtle (Backhousia citriodora), native thyme (Prostanthera incisa), sea parsley (Apium prostratum), seablite (Suaeda australis) and saltbush (Atriplex cinerea). Some of the secondary metabolites from these plants exhibit significant antioxidant activity and enzyme inhibition, like α-amylase inhibition. Therefore, these herbs may be preventive not only against cardiovascular diseases but also type 2 diabetes. The enzyme α-amylase breaks down polysaccharides, thereby increasing blood sugar levels. Recent studies suggest that hyperglycemia induces generation of reactive oxygen species, alteration of endogenous antioxidants and oxidative stress. It was found that patients with uncontrolled sugar levels in addition to diabetes also suffer from accelerated cognitive decline independent of their age. Although Australian native herbs are used as a substitute for related European plants, their medicinal properties are much less studied.
After preparing the extracts, the scientists began to study their composition and qualities. Rosemary and oregano extracts showed the greatest antioxidant activity, while sage, oregano and thyme were the best at slowing down reactions involving α-amylase (extracts from lavender flowers and leaves were the only ones not to show this effect). Among the studied Australian native herbs, lemon myrtle showed the strongest antioxidant properties, with the best α-amylase inhibition observed with extracts of native thyme (this property was noticed for the first time), sea parsley and saltbush.
The study of plant extracts using bioassay and thin-layer chromatography allows scientists to examine a variety of compounds, find mixtures that have the desired properties and isolate substances that exhibit them to the greatest extent. This fast and cost-effective method will be useful for finding new drug compounds.
Read the papers: Applied Sciences, Journal of Pharmaceutical and Biomedical Analysis and Journal of Chromatography A.
Article source: Sechenov University via Eurekalert
Image credit: Melburnian / Wikimedia
A group of cell biologists has reveal in a new study that the phytochrome B molecule has unexpected dynamics activated by temperature, and behaves differently depending on the temperature and type of light.
A protein hijacked from a bacterial pathogen helps to facilitate more precise genome editing in plants. A new genome editing system enhances the efficiency of an error-free DNA repair pathway, which could help improve agronomic traits in multiple crops.
Staying on top of these collections is time-consuming during the best of times, and this task becomes even more complex in the age of social distancing. Yet thousands of scientists across the globe are doing just that, maintaining everything from crickets, to tissue cultures, mice, powdery mildews, nematodes, psyllids, zebrafish and even rust fungi.
Glyphosate is a widely used broad-spectrum herbicide that targets both broadleaf plants and grasses (dicots and monocots). This recent work aids our understanding of adaptive evolution in amaranth plants and has implications for optimizing pesticide use in the environment.
Unless it happens to be allergy season, most people don’t give a lot of thought to pollen. But new research might change the way we look at a field of flowers. A study suggests that pollen color can evolve independently from flower traits, and that plant species maintain both light and dark pollen because each offers distinct survival advantages.