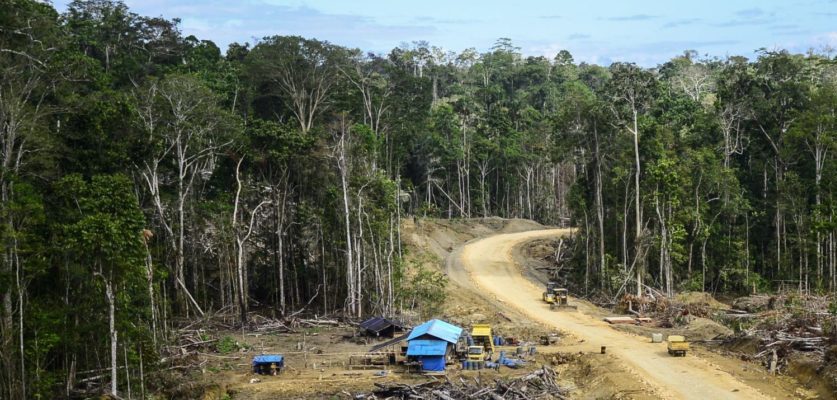
The global COVID-19 pandemic has shed light on the importance of certain previously little-studied scientific areas such as the relationships between ecosystems, their biodiversity and the emergence of new infectious diseases.
The global COVID-19 pandemic has shed light on the importance of certain previously little-studied scientific areas such as the relationships between ecosystems, their biodiversity and the emergence of new infectious diseases.
Scientists have identified five Romaine lettuce varieties that both brown less quickly after fresh-cut processing and are slower to deteriorate postharvest. They also are determining the genetic basis for deterioration.
The native guava is one of the first Australian plants to be pushed to the brink of extinction by a fungal plant disease which has spread rapidly across the globe, according to a new study.
Plant leaves exhibit a great diversity of forms that can be grouped into two types: simple leaves with a single blade and compound leaves with multiple units termed leaflets. A major question for plant developmental biologists is the molecular mechanism underlying diversity of compound leaf form during evolution.
A team of researchers has developed an innovative software program for the simulation of breeding programmes. The “Modular Breeding Program Simulator” (MoBPS) enables the simulation of highly complex breeding programmes in animal and plant breeding and is designed to assist breeders in their everyday decisions.
To make climate scenarios work for decision-makers, an international team of researchers developed a comprehensive interactive online platform. It is the first of its kind to provide the tools to use those scenarios – from climate impacts to mitigation and energy options – to a broader public beyond science. The scenarios help policy makers and businesses, finance actors and civil society alike to assess the threat of global warming and ways to limit it.
A webtool giving an overview of climate change in Europe and predicting subsequent developments was created as a joint collaboration between French, Spanish, German and Estonian researchers.
Some plants, like soybean, are known to possess an innate defense machinery that helps them develop resistance against insects trying to feed on them. However, exactly how these plants recognize signals from insects has been unknown until now. In a new study, scientists have uncovered the cellular pathway that helps these plants to sense danger signals and elicit a response, opening doors to a myriad of agricultural applications.
Plants produce the hormone jasmonic acid as a defence response when challenged. This is how they ensure that their predators no longer like the taste of their leaves. Biologists want to find out whether biological precursors and other variants of jasmonic acid lead to similar or different effects. But such derivatives of the hormone have so far been too expensive for experiments and difficult to come by. Researchers have now found a method that might make the production of a biologically significant precursor of jasmonic acid more efficient and cheaper.
By manipulating the expression of one gene, geneticists can induce a form of “stress memory” in plants that is inherited by some progeny, giving them the potential for more vigorous, hardy and productive growth, according to researchers, who suggest the discovery has significant implications for plant breeding. And because the technique is epigenetic — involving the expression of existing genes and not the introduction of new genetic material from another plant — crops bred using this technology could sidestep controversy associated with genetically modified organisms and food.