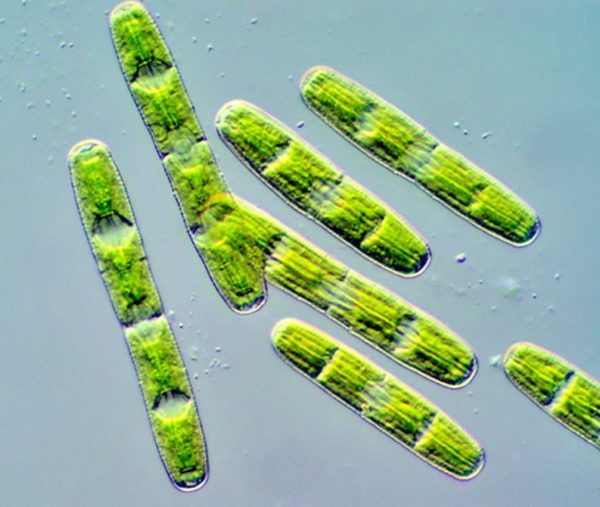
Researchers have sequenced and analyzed the genome of a single-celled alga that belongs to the closest lineage to terrestrial plants and provides many clues to how aquatic plants first colonized land.
Researchers have sequenced and analyzed the genome of a single-celled alga that belongs to the closest lineage to terrestrial plants and provides many clues to how aquatic plants first colonized land.
A new study is calling for governments to reallocate subsidies to encourage the use of lower risk control options – such as biopesticides – in the fight against the devastating maize pest fall armyworm (FAW).
Over 80% of the world’s flowering plants must reproduce in order to produce new flowers, according to the U.S. Forest Service. This process involves the transfer of pollen between plants by wind, water or insects called pollinators — including bumblebees. In a new study, researchers discovered a spiny pollen that has evolved to attach to traveling bumblebees.
Researchers have discovered a new species of seaweed Calidia pseudolobata as well as four new genera of red algae from the warm waters of China. The research suggests there are many new species yet to be discovered – with potential implications for marine biodiversity and food security.
The global COVID-19 pandemic has shed light on the importance of certain previously little-studied scientific areas such as the relationships between ecosystems, their biodiversity and the emergence of new infectious diseases.
Scientists have identified five Romaine lettuce varieties that both brown less quickly after fresh-cut processing and are slower to deteriorate postharvest. They also are determining the genetic basis for deterioration.
The native guava is one of the first Australian plants to be pushed to the brink of extinction by a fungal plant disease which has spread rapidly across the globe, according to a new study.
Plant leaves exhibit a great diversity of forms that can be grouped into two types: simple leaves with a single blade and compound leaves with multiple units termed leaflets. A major question for plant developmental biologists is the molecular mechanism underlying diversity of compound leaf form during evolution.
A team of researchers has developed an innovative software program for the simulation of breeding programmes. The “Modular Breeding Program Simulator” (MoBPS) enables the simulation of highly complex breeding programmes in animal and plant breeding and is designed to assist breeders in their everyday decisions.
To make climate scenarios work for decision-makers, an international team of researchers developed a comprehensive interactive online platform. It is the first of its kind to provide the tools to use those scenarios – from climate impacts to mitigation and energy options – to a broader public beyond science. The scenarios help policy makers and businesses, finance actors and civil society alike to assess the threat of global warming and ways to limit it.