Carolina Roa, Independent Consultant at CropIP
“We need a material transfer agreement”
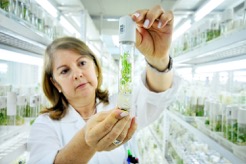
© CIAT, licensed under Creative Commons CC BY-SA.
As a plant breeder in the area of food and agriculture you look for well-characterized – or at least well-referenced – plant materials suitable for making crosses and generating populations to be tested for agricultural traits. If you or your organization don’t already have such materials, you are likely to contact people at seed or germplasm banks, research or breeding programs to obtain sexual or vegetative seeds.
Have the entities come back to you saying that to get access to the plant material you and/or your organization need to agree to the terms of a material transfer agreement (MTA)? Have they perhaps used the expression “Standard Material Transfer Agreement (SMTA)”? Likewise, if you wanted to provide germplasm to a colleague or a breeder/researcher at another institute, has your own organization told you that an MTA or an SMTA is required? You may be asking yourself, “What is an MTA or SMTA, and why are they required?” This article aims to address these questions.
International and national contexts behind the agreements
Around 20 years ago, no written agreement was necessary to exchange plant materials used for research, breeding or training in the area of food and agriculture, particularly if one was working in the public sector. A verbal agreement was likely to suffice. The latter, however, meant that access to plant materials depended in great measure on personal or inter-organizational relationships, geographic proximity, reciprocity and mutual gain, and interactions between governments1.
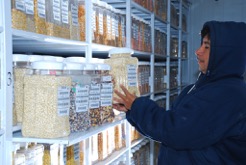
Maize active collection. © Xochilquetzal Fonseca, CIMMYT, licensed under Creative Commons CC BY-NC-SA.
In the early 1990s the situation changed with the advent of two major international treaties. The Convention on Biological Diversity (CBD), in force since 1993, deals with access to all biological diversity, including all genetic resources, as well as the sharing of benefits arising from their use. The International Treaty on Plant Genetic Resources for Food and Agriculture (ITPGRFA; referred to as the Plant Treaty), in force since 2004, carved a niche for plant genetic resources for food and agriculture (PGRFA) and created a multilateral system to facilitate access and benefit sharing for PGRFA deemed important for food security2.
A large number of countries are members of one or both of these treaties, currently 194 countries in the case of the CBD and 134 in the case of the Plant Treaty. The country members (called Contracting Parties) have implemented national laws and regulatory measures to adopt and adapt these regimes at national levels. Implementation, however, is not uniform. Some countries have amended existing national laws to incorporate the main aspects of the international treaties. Others have issued specific national laws that reproduce the international instruments and have added aspects pertinent to their national contexts, and a number of countries have not yet implemented the treaties at a national level. Therefore, as a plant breeder/researcher you are likely to encounter different rules and conditions for accessing or providing PGRFA, depending on whether the country where the materials are located have implemented the CBD, the Plant Treaty, both or none, and depending on the rules and regulations applicable at the organizations hosting/administering the plant materials, including your own institute.
MTA or SMTA? What’s the difference?
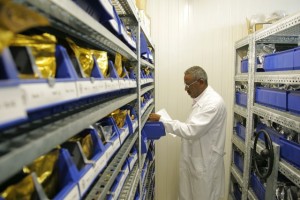
ILRI forage genebank © Stevie Mann, ILRI, licensed under Creative Commons CC BY-NC-SA.
Whether an MTA with specific conditions of access and use, or the SMTA with standardized conditions applies for the plant materials to be exchanged depends on whether CBD-derived regulations apply, or whether the Plant Treaty operates. In case CBD rules apply, you or your institute, as a prospective recipient, will receive an MTA with conditions defined by the germplasm provider. You/your institute will need to accept the terms as they are, or try negotiating and modifying them to suit your purposes. This process normally takes time and legal skills. Going through this bilateral negotiation process every time new plant material is requested from another entity could be a deterrent to the research, and breeding work might not progress at the pace and scale that is needed to address growing food security challenges.
The SMTA, as its name indicates, was designed by the negotiators of the Plant Treaty as a standard and multilateral MTA with fixed terms and conditions of access and use, applicable to plant materials from 64 food and feed crops listed in Annex 1 of the Plant Treaty, which are under public management and control and in the public domain. This system is referred to as the Multilateral System of access and benefit sharing (MLS for short). The SMTA also applies to PGRFA placed voluntarily into the MLS by its holders. Therefore, if particular PGRFA required for research, breeding and/or training purposes are under the MLS, the SMTA as it is applies without the need to negotiate terms, saving time and costs.
At this point it is worth clarifying that PGRFA, even belonging to the crops listed in Annex 1 of the Plant Treaty, owned or administered by private corporate entities are generally outside the MLS. Likewise, PGRFA of the listed crops growing in farmers’ fields, or PGRFA under development by breeders or farmers (that is, not ready for commercialization and commonly referred to as ‘breeding materials’) may not be under the MLS. Their inclusion in the MLS is at the discretion of the owner/holder, the grower or the developer of the breeding materials. If they place such materials under the MLS, they will need to use the SMTA as the instrument for access and benefit sharing for the purposes specified in the Plant Treaty. However, the developer is entitled to add terms and conditions to the SMTA.
How the SMTA works3
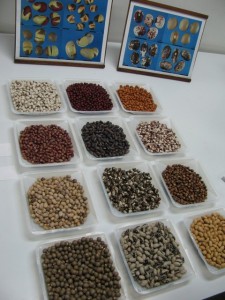
CIAT genebank © Luigi Guarino, licensed under Creative Commons CC BY.
Scope of use – PGRFA under the SMTA can be used for research, breeding and/or training in the fields of food and agriculture. If the intended use is different, e.g., extraction of compounds to be used for chemical or pharmaceutical applications, the SMTA is not the instrument to use. Other conditions dictated by national legislation, the holder/owner of the resources, or both may apply for non-food/feed applications.
Facilitated access – access to PGRFA should be free of charge and expeditious, without the need to track individual accessions. If a fee is charged, it should reflect ‘minimal costs’ related to shipment and transport costs. For instance, costs of seed maintenance, seed production, and the like should not be included.
Provider’s obligations and rights – the main obligations of PGRFA providers include (1) granting ‘facilitated access’ to PGRFA and associated passport data and non-confidential descriptive information, and (2) reporting periodically to the Secretariat of the Plant Treaty about the SMTAs entered into. As a provider and developer of breeding materials, you will have discretion on granting access to such materials while they are under development. If you grant others access to such materials, you’d be entitled to add terms and conditions to the SMTA, including aspects such as payments, limitations on subsequent transfers, etc.
Recipient’s obligations and rights – the main obligations that come with materials received under the SMTA include: (1) to exclusively use them for research, breeding, and/or training related to food and agriculture; (2) to not claim intellectual property rights or any other rights that may limit facilitated access; (3) to use a new SMTA for subsequent transfers; and (4) to report such subsequent transfer to the Secretariat of the Plant Treaty.
If the recipient were to subsequently transfer PGRFA under development, the recipient will act as a provider and in this case, s/he should (1) use a new SMTA; (2) identify in Annex 1 of the new SMTA the material from which the breeding materials were derived; and (3) report this transaction to the Secretariat of the Plant Treaty.
If additional conditions are added to the SMTA for the transfer (or the subsequent transfer) of PGRFA under development, they should go as a separate agreement to the associated SMTA and there is no need to report such add-on conditions to the Secretariat of the Plant Treaty. A recipient of PGRFA, whether under development or not, has no further duties with respect to the actions of a subsequent recipient.
Benefit sharing commitments – As a recipient, you are expected to share the benefits obtained from MLS materials with the agricultural community in general. As an example, granting access for further research and breeding to products developed by incorporating MLS materials received, is one of such benefits. In this case, you may also voluntarily contribute funds to the Benefit Sharing Fund, administered by the Plant Treaty, which finances food and feed-related research projects, mostly in developing economies. Conversely, if you decided to restrict further access to your MLS-derived products, you would be required to pay to the Benefit Sharing Fund either 0.77% or 0.5% of the sales of your product, depending on whether you opted to pay per accession received (first amount) or per crop accessed (second figure). The payment requirement operates regardless of how much MLS-derived material has been incorporated into your product and it will last as long as access to the product is restricted.
Duration – the particular SMTA you entered into will be valid as long as the Plant Treaty remains in force.
Genebanks using the SMTA
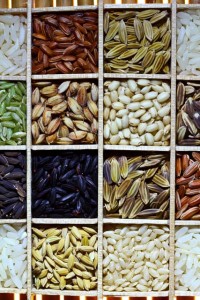
Rice seed varieties. © IRRI, licensed under Creative Commons CC BY-NC-SA 2.0.
Apart from national seed collections of member countries, there are international institutions that have placed their seed holdings under the purview of the Plant Treaty. The International Agricultural Research Centers of the Consultative Group on International Agricultural Research (CGIAR) are among those institutions. Eleven of the CGIAR centers, holding approximately 700,000 accessions of crops listed in Annex 1, as well as non-Annex 1 crops and breeding materials, use the SMTA to transfer these materials for the purposes specified by the Plant Treaty.
The individual websites of the CGIAR centers publish lists of available accessions, and requests can be placed electronically. As a prospective recipient, you should receive confirmation of availability of sufficient seed for shipping together with an electronic copy of the SMTA. You have the options to accept the SMTA terms through a mouse click, by signature, or by ripping the package containing the seed and a printed copy of the SMTA. From this point onwards, the rights and obligations of the SMTA for both providers and recipients start operating.
Therefore, next time you receive an SMTA don’t despair; come back to these notes and seek guidance from the legal or other pertinent office at your organization on how to proceed with this or any other kind of agreement. ©
REFERENCES
- Halewood, M (2013). What kind of goods are plant genetic resources for food and agriculture? Towards the identification and development of a new kind of commons. International Journal of the Commons 7(2): 278–312.
- Moore, Gerard and Tymowski. 2005. Explanatory guide to the International Treaty on Plant Genetic Resources for Food and Agriculture. IUCN, Gland, Switzerland and Cambridge, UK. xii + 212 pp.
- Standard Material Transfer Agreement (accessed at http://www.planttreaty.org/content/what-smta).
About the author: Carolina Roa is plant biologist and a legal professional with around 25 years of experience. She has worked for the public and private sector in different parts of the world on a range of legal and intellectual-property aspects related to agriculture and biotechnology. Carolina is currently the Principal Consultant at CropIP. She can be reached at carolina@crop-ip.info.