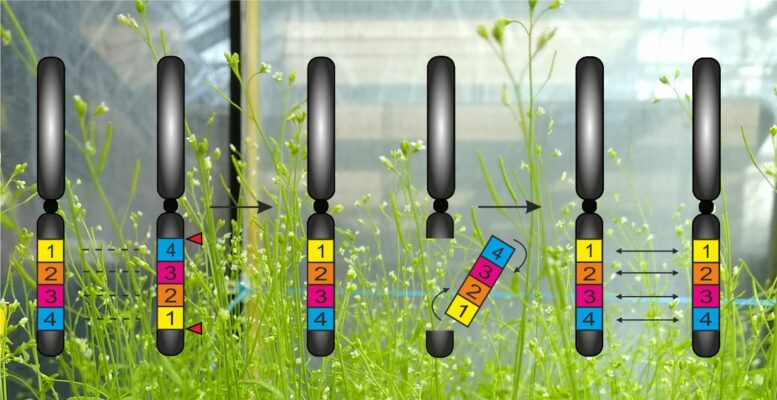
For the First Time, Researchers Use CRISPR/Cas Molecular Scissors to Recombine Genes on a Chromosome. A new application of the CRISPR/Cas molecular scissors promises major progress in crop cultivation.
For the First Time, Researchers Use CRISPR/Cas Molecular Scissors to Recombine Genes on a Chromosome. A new application of the CRISPR/Cas molecular scissors promises major progress in crop cultivation.
An international team has decoded the full genome for the black mustard plant—research that will advance breeding of oilseed mustard crops and provide a foundation for improved breeding of wheat, canola and lentils.
Reading the Basmati Genome Provides Clues for Growing Drought-Tolerant and Bacteria-Resistant Rice. Using an innovative genome sequencing technology, researchers assembled the complete genetic blueprint of two basmati rice varieties, including one that is drought-tolerant and resistant to bacterial disease.
With the help of new genomic sequencing and assembly tools, plant scientists can learn more about the function and evolution of highly destructive plant pathogens that refuse to be tamed by fungicides, antibacterial, and antivirals.
An international group of researchers has assembled the most complete genome sequence of commercial sugarcane. They mapped 373,869 genes or 99.1% of the total genome.
Researchers have developed an improved assembly of the genome for the date palm using long-read sequencing technology. This improvement over the current versions of the genome will help advance further research, and also inform the propagation practices of this essential MENA region food source.
Oaks have a complex evolutionary history that has long eluded scientists. New research, however, provides the most detailed account to date of the evolution of oaks, recovering the 56-million-year history that has made the oaks one of the most diverse, abundant and important woody plant groups to the ecology and economy of the northern hemisphere.
An international team succeeded in assembling the first sequence of the pea genome. This study will, in addition to increasing knowledge of this genome compared to that of other legumes, help to improve traits of interest for peas, such as disease resistance, regularity of yield and nutritional value.
This week’s post was written by Jonathan Ingram, Senior Commissioning Editor / Science Writer for the Journal of Experimental Botany. Jonathan moved from lab research into publishing and communications with the launch of Trends in Plant Science in 1995, then going on to New Phytologist and, in the third sector, Age UK and Mind.
In this week of the XIXth International Botanical Congress (IBC) in Shenzhen, it seems appropriate to highlight outstanding research from labs in China. More than a third of the current issue of Journal of Experimental Botany is devoted to papers from labs across this powerhouse of early 21st century plant science.
Collaborations are key, and this was a theme that came up time again at the congress. The work by Yongzhe Gu et al. is a fine example, involving scientists at four institutions studying a WRKY gene in wild and cultivated soybean: in Beijing, the State Key Laboratory of Systematic and Evolutionary Botany at the Institute of Botany in the Chinese Academy of Sciences, and the University of the Chinese Academy of Sciences; and in Harbin (Heilongjiang), the Crop Tillage and Cultivation Institute at Heilongjiang Academy of Agricultural Sciences, and the College of Agriculture at Northeast Agricultural University. Interest here centers on the changes which led to the increased seed size in cultivated soybean with possible practical application in cultivation and genetic improvement of such a vital crop.
Botanic gardens are also part of the picture. In another paper in the same issue, Yang Li et al. from the Key Laboratory of Tropical Plant Resources and Sustainable Use at Xishuangbanna Tropical Botanical Garden in Kunming (Yunnan) and the University of the Chinese Academy of Sciences in Beijing present research on DELLA-interacting proteins in Arabidopsis. Here the authors show that bHLH48 and bHLH60 are transcription factors involved in GA-mediated control of flowering under long-day conditions.
Naturally, research on rice is important. Wei Jiang et al. from the National Key Laboratory of Crop Genetic Improvement, Huazhong Agricultural University (Wuhan) describe their research on WOX11 and the control of crown root development in the nation’s grain of choice, which will be important for breeders looking to increase crop yields and resilience.
The other work featured is either in Arabidopsis or plants of economic importance: Fangfang Zheng et al. (Qingdao Agricultural University, also with collaborators in Maryland) and Xiuli Han et al. (Beijing); Yun-Song Lai et al. (Beijing/Chengdu – cucumber), Wenkong Yao et al. (Yangling, Shaanxi – Chinese grapevine, Vitis pseudoreticulata), and Xiao-Juan Liu et al. (Tai-an, Shandong – apple).
Shenzehn has grown rapidly and is now highly significant for life science as home to the China National GeneBank (CNGB) project led by BGI Genomics. The vision as set out by Huan-Ming Yang, chairman of BGI-Shenzhen, is profound – from sequencing what’s already here, often in numbers per species, to innovative synthetic biology.
Shenzehn is also home to another significant institution, the beautiful and scientifically important Fairy Lake Botanic Garden. At the IBC, the importance of biodiversity conservation for effective, economically focused plant science, but also for so many other reasons to do with our intimate relationship with plants and continued co-existence on the planet, was a central theme.
The research highlighted in Journal of Experimental Botany is part of the wider, positive growth of plant science (and, indeed, botany) not just in China, but worldwide. The Shenzehn Declaration on Plant Sciences with its seven priorities for strategic action, launched at the congress, will be a guide for the right development in coming years.
Professor Henk Hilhorst (Wageningen University and Research)
This week we spoke to Professor Henk Hilhorst (Wageningen University and Research) about his research on desiccation tolerance in seeds and plants.
Could you begin by telling us a little about your research?
I am a plant physiologist specializing in seed biology. I have a long research record on various aspects of seeds, including the mechanisms and regulation of germination and dormancy, desiccation tolerance, as well as issues in seed technology. Being six years from retirement now, I decided to extend my desiccation tolerance studies from seeds to resurrection plants, which display vegetative desiccation tolerance. I strongly believe that unveiling of the mechanism of vegetative desiccation tolerance may help us create crops that are truly tolerant to severe drought, rather than (temporarily) resistant.
How did you become interested in this field of study, and how has your career progressed?
As with many things in life, it was coincidence. I majored in plant biochemistry and applied for a PhD position in seed biology. After obtaining the degree I was offered a tenure track position in seed physiology by the Laboratory of Plant Physiology at Wageningen University, where I still work as a faculty member. My career has progressed nicely and I am an authority in the field of seed science, editor-in-chief of the journal Seed Science Research, and will become the President of the International Society for Seed Science in September of this year.
I see my current work on vegetative desiccation tolerance as a highlight in my professional life. I have always been more interested in the desiccation tolerance of seeds until about five years ago, when my current collaborator Prof Jill Farrant of the University of Cape Town, South-Africa, made me enthusiastic about these wonderful resurrection plants. We started to work together and published our first study recently in Nature Plants.
Read the paper here ($): A footprint of desiccation tolerance in the genome of Xerophyta viscosa.
In your recent paper, you sequenced the genome of the resurrection plant, Xerophyta viscosa, which can survive with less than a 5% relative water content. How is it possible for a plant to lose so much of its water and still survive?
These plants have a lot of characteristics that we’ve seen in seeds. They display protective desiccation tolerance mechanisms in their leaves, including anti-oxidants, protective proteins, and even dismantle their photosynthetic machinery during periods of drought. Even the cell wall structure and composition of resurrection plants resemble those of seeds. We are currently working on a paper describing the striking similarities between seeds and resurrection plants.
What was the most interesting discovery you made upon sequencing the genome of the resurrection plant?
First, the similarities between resurrection plants and seeds listed above were also apparent at the molecular level. For example, previous work suggested that the “ABI3 regulon”, consisting of about 100 genes regulated by the transcription factor ABI3, is specific to seeds, but we found that it is almost completely present (and active) in the leaves of Xerophyta viscosa too!
Secondly, we found “islands” or clusters of genes specific for desiccation tolerance that aren’t found in other species. Many of these regulate secondary metabolite pathways.
How challenging was it to sequence the genome of this plant? How did you overcome any difficulties?
It was very challenging. First, the species is an octoploid, meaning it has eight copies of each chromosome. This meant that we had to sequence its genome at very high coverage and employing the most advanced sequencing facilities, e.g. PacBio. Getting funding for this complex analysis was another challenge. We then took almost a year to assemble the genome and annotate it at the desired quality.
You identified some of the most important genes involved in desiccation tolerance. Is it possible to translate this work into other species, such as crops that may be threatened by drought as the climate changes?
That will be our ultimate goal. It’s important to remember that desiccation-sensitive plants, including all our major crops, produce seeds that are desiccation tolerant. This implies that the information for desiccation tolerance is present in the genomes of these crops but that it is only turned on in the seeds. We are trying to determine how this is localized, in order to find a method to turn on the desiccation tolerance mechanism in vegetative parts of the (crop) plant too. In parallel we are expressing some of the key transcription factors from Xerophyta viscosa in some important crops to see how this affects them.
Are there any other interesting aspects of Xerophyta viscosa biology?
Contrary to plants that wilt and ultimately die because of (severe) drought, leaves of resurrection species do not show such stress-related senescence. This is related to the engagement of active anti-senescence genes during the drying of the leaves of resurrection species. We are currently investigating these senescence-related mechanisms too.
The rose of Jericho (Anastatica hierochuntica) is another resurrection plant. Image credit: FloraTrek. Used under license: CC BY-SA 3.0.
Do you expect to find that different types of desiccation-tolerant plants use the same subset of genes to survive drought, or could they have developed other pathways to resilience?
We expect that the core mechanism is very similar among the resurrection species but that each species may have adapted to its specific environment.
Funding permitting, we will sequence the genomes of at least another ten resurrection species to further clarify the various evolutionary pathways to desiccation tolerance and, importantly, to discriminate between species-specific and desiccation tolerance-specific genes.
What advice do you have for early career researchers?
Stick to what you believe in, even if you have to (temporarily) be involved in research that you appreciate less, e.g., because of better funding opportunities.
Read Henk’s recent paper in Nature Plants here ($): A footprint of desiccation tolerance in the genome of Xerophyta viscosa.