Author: Chandan Gautam is a Postdoctoral Fellow researcher based at Institute of Plant and Microbial Biology, Academia Sinica, Taiwan.
To enable fast, efficient and cost effective bioengineering of plants, new tools and methods to deliver the genetic material into plant cells are increasingly being researched. Nanoparticles assisted delivery of biomolecules is one such under explored tool for their application in plant system. Nanoparticles could offer viral-vector free delivery platform with low toxicity, high delivery efficiency, capability of subcellular targeting, with ability to undergo diverse cargo conjugation and delivered with minimal or no physical or chemical aids into broad range of hosts (Jat et al., 2020).
Nanomaterials like DNA nanostructures, carbon nanotubes, magnetic nanoparticles, mesoporous silica nanoparticles and carbon nanotubes have been successfully demonstrated to deliver nucleic acids into plant cells (Chang et al., 2013; Kwak et al., 2019; Zhang et al., 2019; Zhang et al., 2020). A better understanding of nanoparticle–plant interactions is perquisite to enable the design of nanoparticle delivery platforms for the modification of plant physiology to produce desired traits. The rigid plant cell walls is a major challenge for successful delivery of biomolecules inside the cell. Nanoparticles can circumvent this challenge and thus are emerging as preferred delivery vehicles in plants (Jat et al., 2020).
In their recent paper in Plant Physiology, Yong et al., (2021) have demonstrated the use of layered double hydroxide (LDH) nanoparticles to successfully delivery double stranded RNA (dsRNA) into early bicellular pollen of tomato (Solanum lycopersicum). LDHs are synthetic clays that contain cationic layers with anions and water in the interlayer for charge balance (Shanmuganathan and Ellison, 2014). Owing to their lamellar structure and positive charge, LDH nanoparticles act as a suitable carrier to deliver negatively charged biomolecules, including nucleic acids and proteins (Xu et al., 2007; Zhang et al., 2021). In the current study, LDH nanoparticles were synthesised, and characterized using transmission electron microscopy (TEM), X-ray diffraction (XRD) and Fourier-transform infrared (FTIR) spectrum analysis. To study internalization of LDH nanoparticles into the pollen cells, they were labelled with fluorescein isothiocyanate (FITC) which produces green fluorescence. The LDH with size of under 50 nm (LDH-50) were incorporated more effectively inside the early bicellular pollens (compared to other stages of pollen development) as determined by both fluorescent intensity from flow cytometry data and confocal microscopy images.
After finalizing the LDH particle size and pollen stage for effective transfection, LDH-50 capability to facilitate the uptake of nucleic acid (dsRNA) was investigated. For this purpose, Cucumber Mosaic Virus dsRNA labelled in vitro with Cy3 (orange-fluorescent dye) (dsRNA-Cy3), was loaded onto LDH-50. Following incubation of LDH-50: dsRNA-Cy3 with pollen cells, effective enhancement in the internalization of dsRNA was observed using fluorescent imaging. Next, they validated the functionality and efficiency of this platform by delivering GUS (β-glucuronidase) dsRNA via LDH-50 to silence the GUS reporter gene in the developing pollen from the transgenic line sAc 10512i GUS homozygotes (Keddie et al., 1998). GUS activity and mRNA levels were measured to analyse the effectiveness of gene silencing. For the measurement of GUS activity, the early bicellular pollens were subjected to a modified fluorometric 4-methylumbelliferyl ß-D-glucuronide (MUG) assay, wherein MUG is converted into the fluorescent product 4-methylumbelliferone (MU) by ß –glucuronidase, as indication of GUS activity. The LDH-50–dsRNA was successfully able to induce GUS activity inhibition in a time-dependent process. However, as the inhibition of GUS activity is an indirect indicator of GUS-transgene silencing, and is dependent on the stability of GUS protein; quantification of GUS mRNA levels was carried out in pollens incubated with naked GUS dsRNA or GUS dsRNA-LDH-50 complex to further validate the effectiveness of gene silencing. Incubation with GUS dsRNA-LDH-50 complex significant reduced GUS mRNA levels compared with the untreated GUS-positive control pollen and naked GUS dsRNA treatment, thus establishing the effectiveness of this platform. The schematic illustration of LDH nanoparticles facilitating pollen uptake of dsRNA has been shown in Figure 1.
Concisely, the authors described targeted gene silencing using LDH nanoparticles and thoroughly investigated factors (like particle size, the dsRNA dose, the LDH–dsRNA complexing ratio, and the treatment time) affecting the efficiency of this nucleic acid delivery platform. These findings present a simple biomolecule delivery tool for engineering plant cell systems for trait manipulation, including metabolic engineering and RNA silencing-based improvement of antiviral immunity in plants.
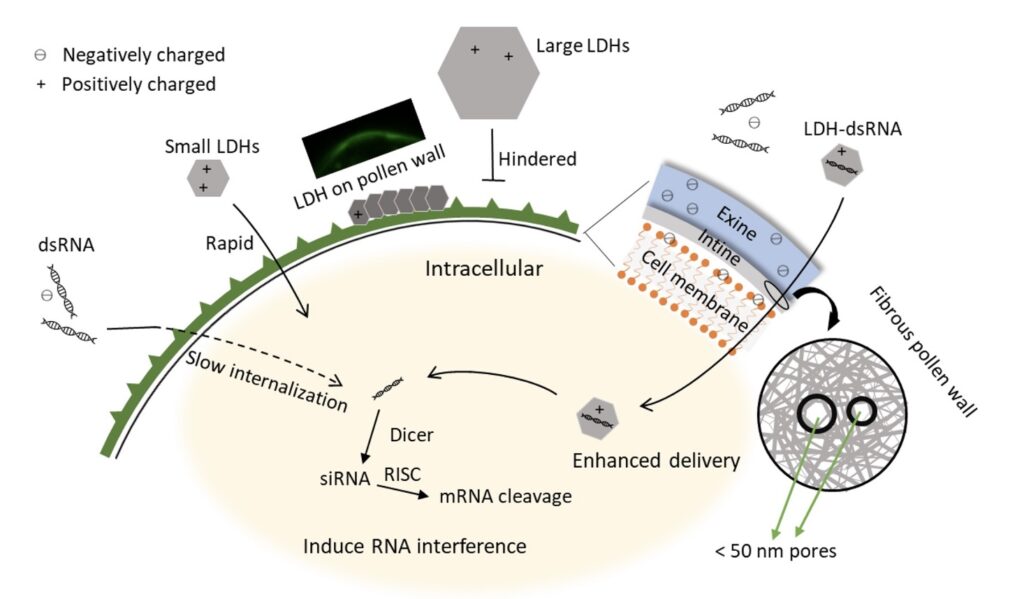
References
- ChangFP, KuangLY, HuangCA, JaneWN, HungY, HsingYIC, MouCY (2013) A simple plant gene delivery system using mesoporous silica nanoparticles as carriers.Journal of Materials Chemistry B 1: 5279–5287 doi.org/10.1039/C3TB20529K
- JatSK, BhattacharyaJ, SharmaMK (2020) Nanomaterial based gene delivery: A promising method for plant genome engineering.Journal of Materials Chemistry B 8: 4165–4175 doi.org/10.1039/D0TB00217H
- KeddieJS, CarrollBJ, ThomasCM, ReyesMEC, KlimyukV, HoltanH, GruissemW, JonesJDG (1998) Transposon tagging of the Defective embryo and meristems gene of tomato.Plant Cell 10: 877–887 doi.org/10.1105/tpc.10.6.877
- KwakSY, LewTTS, SweeneyCJ, KomanVB, WongMH, Bohmert-TatarevK, SnellKD, SeoJS, ChuaNH, StranoMS (2019) Chloroplast-selective gene delivery and expression in planta using chitosan-complexed single-walled carbon nanotube carriers.Nature Nanotechnology 14: 447–455 doi.org/10.1038/s41565-019-0375-4
- ShanmuganathanK, EllisonCJ (2014) Layered Double Hydroxides: An Emerging Class of Flame Retardants.Polymer Green Flame Retardants. Elsevier Inc., pp 675–707 doi.org/10.1016/B978-0-444-53808-6.00020-2
- XuZP, WalkerTL, LiuK, CooperHM, LuGQM, BartlettPF (2007) Layered double hydroxide nanoparticles as cellular delivery vectors of supercoiled plasmid DNA.International journal of nanomedicine 2: 163–74 www.ncbi.nlm.nih.gov/pmc/articles/PMC2673978/
- YongJ, ZhangR, BiS, LiP, SunL, MitterN, CarrollBJ, XuZP (2021) Sheet-like clay nanoparticles deliver RNA into developing pollen to efficiently silence a target gene.Plant Physiology 1–14 doi.org/10.1093/plphys/kiab303
- ZhangH, ZhangH, DemirerGS, González-GrandíoE, FanC, LandryMP (2020) Engineering DNA nanostructures for siRNA delivery in plants.Nature Protocols 15: 3064–3087 doi.org/10.1038/s41596-020-0370-0
- ZhangLX, HuJ, JiaYB, LiuRT, CaiT, XuZP (2021) Two-dimensional layered double hydroxide nanoadjuvant: Recent progress and future direction.Nanoscale 13: 7533–7549 doi.org/10.1039/D1NR00881A
- ZhangR, MengZ, AbidMA, ZhaoX (2019) Novel pollen magnetofection system for transformation of cotton plant with magnetic nanoparticles as gene carriers.Methods in Molecular Biology. pp 47–54 doi.org/10.1007/978-1-4939-8952-2_4
Read the paper: Plant Physiology
Author: Chandan Gautam